Colours are part of nature and are all around us, affecting our thoughts and our mood, inspiring us and comforting us.
Clearly, as a species, we have felt that we need to incorporate colours into our lives, whether that is in the form of clothes,
gems, metals or a garden full of flowers. Paintings are a logical extension of this and virtually anything can be painted so how
does this work?
Light can enter our eyes having been reflected and modified by a surface or directly from a coloured light source. For a painting to be seen properly, it should be illuminated by a light source that our eyes can adjust to such as daylight or artificial lights - tungsten, fluorescent, LED lights and so on (unless the art is designed to be viewed under different conditions, such as if it is made using fluorescent pigments and is meant to be viewed under ultraviolet light, for example).
Using reflected light, paintings attempt to reproduce the images that we see in our everyday lives or, in the case of surrealism and related art forms, those that the artist thinks that we should be able to see. There are limits to what you can reproduce in a piece of art but people's eye's adjust to many limits and there are effects that can be used, such as local contrast, to extend the dynamic range of the artist's work.
Black and White
The brightest we can see is white and the darkest we can see is black. Whilst black and white are not achievable as pigments (black reflects some light and white certainly doesn't reflect it all), they do not exist in nature either. The very dark brown-grey pigment that is made from soot, that we use as black in paintings, reflects light in the same way that real soot does. Titanium white pigment is seen as a bright white but it still absorbs some deep blue light and reflects greeny-blue light (500nm) around 5% more than deep red (700nm) so it is far from perfect. However, if a painting has been created using those two colours, our eyes adjust to it and see the colours in between in the same way that if lead white was used as the white (interestingly, lead still slopes down by the same amount towards the red but it doesn't have the blue absorption that titanium oxide does, it carries on reflecting blue, right into the UV so, contrary to popular opinions, lead white actually reflects light better than titanium white throughout the spectrum).
You can see in the picture of the spray from the garden hose catching the light from the low sun and breaking it down into its
colours to form a rainbow (hose-bow?) - showing that sunlight has what is called a continuous spectrum - it pretty much contains
all of the colours that we can see. The process that water uses to split light in this situation is refraction insofar as different
colours are refracted to different extents thus separated - this is called dispersion. However, you can see from the diagram on the
left that light extends in both directions beyond the red into the infrared and in the other direction beyond blue into the ultraviolet
- if you have a camera that is sensitive to these wavelengths and use that in the rainbow experiment, you will see that they are
dispersed in the same way as the visible colours of the spectrum. It is all one big continuum and our eyes can see just a little bit
of it.
Incidentally, whilst our eyes cut off pretty much exactly at 400nm, at the red end of the spectrum, you can see down to around
760nm or so and if you look through an infrared filter, you will see that the grass and leaves are very light and the sky is almost
black although I must caution you to not do this for too long as there is a lot of light going into your eye if you do this and just
because your vision is not particularly sensitive to it does not mean that it cannot do your eye any harm. Another thing that you might
notice is that I say up to 400nm and down to 700nm. Where the values for these numbers might indicate that 400 is smaller than 700, if
I'm talking about something like wavelengths, up and down refer to the energies and light at 400nm has a lot more energy than light at
700nm. There is more about light sources and the way that our eyes see light in the page on Colour Reproduction.
Representing Colours using Absorption
Move the mouse over the colour strips in the diagram on the right to see how the colours that are absorbed and reflected are related to each other.
The main way that pigments and dyes appear coloured is from the absorbing different wavelengths of light to different extents.
As light is electromagnetic with the wavelengths having different amounts of energy, these are absorbed to different extents
according to the electronic configuration of the molecules that form the dyes and pigments. For example, copper salts are capable of
existing in two forms (known as copper I and copper II - these are different oxidation states) and the carbonate salt of each of these
absorbs light differently so copper I carbonate is azurite which is a blue with a slight greenish
tinge (the energy of light in the red to yellow part of the spectrum is just right for azurite to absorb). On the other hand, copper II carbonate is malachite which is green (the energy of light in the red and blue parts of the spectrum is just right for malachite to absorb). Basically, your eyes
see the light that is left over after all of these electronic shenanigans.
There are many chemicals around us that can modify light to make colours - rocks, minerals, plant dyes and even dyes made from
insects and then there are metals such as gold, silver, tin, copper and more recently, palladium and aluminium which, if they are not
used strictly as a pigment in art, their reflective properties are used to reflect the light in a way that is very different to normal
pigments. To qualify as a useful pigment or a dye, it has to: change the light that is shone onto it; not react with its
environment; and last long enough to be useful. However, if you just paint a suspension of pigment in water or other volatile
liquid onto a smooth surface, after a while, a lot of it would probably fall off. Clearly, we need something to hold it in
place, some sort of transparent medium - a glue of some sort.
Then, we need to be able to put the paint on the surface so it needs to be in a form that we can apply. Normally, paints are
quite viscous so they need to have some sort of solvent added to them in order to thin them down so that they can be applied
effectively. The solvent depends upon the medium but for a given solvent type, there are ways of making sure that you get the
best solvent for the job.
Finally, you need to have a surface that you can apply the paint to - known as a ground - and that needs to be on something
that will allow it to be displayed appropriately - the support.
|
|
R e f l e c t e d |
 |
 |
 |
 |
 |
 |
 |
 |
 |
 |
 |
 |
 |
 |
 |
 |
 |
 |
 |
 |
 |
 |
 |
 |
 |
A b s o r b e d |
 |
 |
 |
 |
 |
 |
 |
 |
 |
 |
 |
 |
 |
 |
 |
 |
 |
 |
 |
 |
 |
 |
 |
 |
 |
|
- Pigments - making colours work
- Dyes - organic dyes also make good colours but you can't just mix the dye with the medium
- Impurities - unless you have a synthetic pigment, it will have impurities
- Particle Size - Different particle size in some pigments can change hue or depth of colour
- Depth - In some pigments, grinding the particles finer will just make the colour paler
- Hue - Some colours change their hue according to particle size. How does that work?
- Masstone - Thickness and imperfect colours
- Media - Keeping it all in place
- Pigment Volume Concentration (PVC) - how much pigment is in the medium can effect the way it performs
- Solvents / Thinners - why use solvents and which are better than others?
- Grounds and Supports - The paint has to go on something
- Mixing colours - What happens when you mix colours
Ever since people started painting, they have, in effect, wandered around the surface of this planet, looking for and collecting
interestingly coloured rocks, taking them home and smashing them up into a fine powder and using them. There are plenty of naturally
occurring minerals giving rise to many colours in the artist's palette.
Naturally occurring minerals include;
- cinnabar (red);
- minium (orangey red);
- realgar (orange);
- litharge (peachy yellow);
- orpiment (yellow);
- malachite (green);
- azurite (greenish blue);
- lapis lazuli (a very rich blue);
- hydrocerussite (white); and,
- so on.
It might be worth noting at this stage that realgar and orpiment are both arsenic compounds,
There are also a number of 'earth' colours, one group of which contain amounts of manganese dioxide and various hydration states of
iron oxide ranging from a purplish red, through red and orange to yellow, then there are other earths that are shades of green
(containing chromium compounds) and blue (copper compounds).
Man-made pigments include:
- vermilion (chemically identical to cinnabar but has been made as far back as Egyptian times);
- Egyptian Blue (a copper compound);
- lead white (chemically identical to hydrocerussite);
- lead-tin yellow I;
- lead-tin yellow II;
- carbon black (collected from lamps where the smokey flame deposits carbon);
- verdigris (a cyan colour made from treating copper with acetic acid in the presence of water); and,
- so on.
Since the 15th century, there have been many pigments synthesised and with the discovery of new elements such as cadmium and chromium; and the production of titanium as a white pigment as opposed to a buff-coloured pigment, there is a lot more choice. Examples include:
- french ultramarine (chemically identical to lapis lazuli);
- lead chromate;
- prussian blue; and many more.
Dyes are normally thought of as dying fibres or fabric but they can be used in painting as well. However, where, with a normal pigment,
you would just add it to, say, linseed oil or acacia gum solution, you cannot get away with that. The dye would not be stable in the medium
and the dye could migrate within it and leach or bleed into the ground, other paints layers, nearby areas of paint or varnish - this action
being labelled 'fugitive'. Clearly, dyes need to be stabilised with something that is not going to move around within the paint.
Normally, a fine, inert, colourless compound is used as the target of the dye so it gets dyed and then is used as a normal pigment. The
compound normally used for this is alum. The dyed alum 'pigments' are called 'lakes' which comes from the Indian word 'lakh' which means
100,000 and reflects the large quantity of insects needed to dye a useful quantity of cloth.
Woad or Indigo has been used since Egyptian times and the modern chemical industry equivalent is the colour used to dye denim. You can
obtain woad lake pigment from suppliers today.
Rose madder is another lake pigment that has been around since Egyptian times. One of the properties of rose madder is that it
will fluoresce a 'day-glow orange' if you shine ultraviolet light on it. Inside Egyptian tombs, you can see the rose madder on
the walls by shining ultraviolet light on them. (This was noted once by an explorer, I don't suggest that it is something that
you should try for yourself.)
This dye was used to dye the red army tops although over the years, it fades if left in sunlight - clearly, if it is not, it
will last literally thousands of years. Note that fading in a very short period of time is also called 'Fugitive.'
On the right, you can see two images of the Romy 2017 painting - the top in normal, visible light and the lower, illuminated
by ultraviolet light. You can tell from the 'day-glow' orange areas where the rose madder is in the larger harlequin top and
where it isn't in the cat's toy, even though the reds look very similar - look at the orange-red in the toy's headdress and
at the very similar colour right next to it in the harlequin sleeve right next to it.
There are a number of pigments that fluoresce in a distinctive manner - lead white fluoresces white and curiously, Egyptian
blue fluoresces in the infrared when illuminated by visible light.
Carmine is another lake pigment and comes from a beetle from South America. As South America wasn't discovered by Europeans
until a long time after the beginning of the fifteenth century, its inclusion in paintings from before the continent's European
discovery indicates that either the inclusion of the lake is from a more recent repair to the painting or that the painting was
made after that time.
On the right, you can see some malachite as it occurs naturally. Around a quarter of the way up and roughly 40 per cent of the way
across, you can see a small inclusion of rock. When the malachite is ground up, this impurity is also ground up and unless it is
removed from the pigment by some means or other, it will end up in the paint.
Sometimes, the level of impurities in the pigment is low enough for it not to be a problem. However, when the level is too high,
something needs to be done about it.
The easiest way to end up with pigment that has a low enough level of impurities in it is to select sources that are naturally
low in impurities and, of course, the people who sell these know this and will part you from more of your money for the privilege
but sometimes even these sources still need purifying.
So, how do we purify pigments?
Separation methods break down into two main areas - chemical and physical.
Chemical Methods
You can use chemical methods if you are not
particularly bothered about the state of the particles of pigment that you end up with. Chemical methods include
dissolving the pigment or impurities in water, an acid, alkali, some organic solvent or other.
Examples:
- If the impurities dissolved in acid but the pigment didn't, you could use that to remove the impurities and, you
would retain the structural integrity of the pigment - removal of the copper impurity in stack lead white utilises this.
- If both the impurities and the pigment dissolve in acid and say, the pigment was more soluble than the impurity,
once they have dissolved, you can add alkali to precipitate the impurities out first, filter out the precipitate and
then add the rest of the alkali to precipitate out the pigment. This would have the disadvantage that you would lose
the particle structure of the pigment.
The analogous process would exist for pigments that dissolved in alkalies where
you would add acid to precipitate out the components differentially.
- If the impurities dissolve in an organic solvent but the pigment does not, you can 'wash' out the impurities with the
solvent - again, this preserves the structure of the pigment.
- If both the pigment and the impurities dissolve in an organic solvent that is immiscible with water but the impurities
are acidic (for example, an organic acid), dissolve them in the organic solvent and then add some alkali in water and
shake up then let the two layers separate, take the organic solvent layer and evaporate off the solvent.
There are of course many permutations of the above but that should give you an idea of what can be done.
Physical Methods
These methods rely upon differentiating between the pigment and its impurities by physical properties such as density,
whether they are hydrophylic or hydrophobic and so on.
In the photograph on the right, you can see powdered malachite in water. The malachite has tended to settle towards the
bottom of the Erlenmeyer flask and the impurities have tended to stay suspended in the water. Here, the malachite has a greater
density so it sinks first. If the water is tipped off and this procedure repeated, reasonably pure malachite can be obtained.
This separation method depends upon the densities of the pigment and the impurities remembering that if they
both sink, you can make the water denser using a soluble salt such as sodium chloride until you get the best
separation. When they are very similar to each other, the rate at which the particles fall through the liquid
becomes a factor. That can be determined by the shape of the particles when they are ground up insofar as if,
say the pigment particles tend to form roundish particles but the impurities tend to be flat, they will sink at
different rates and then it is a matter of selecting the best time to separate them.
However, there is a limit to this because some malachite stays in suspension with the impurities and some of the impurities
sink with the malachite. Over several purification steps, you can decide how much malachite you are prepared to discard for the
sake of getting an appropriate level of purity in the finished pigment.
You don't have to tip the impurity-rich layer down the sink, you can keep hold of it and in the case of malachite, you can
separate off the water and then dissolve the copper carbonate in acetic acid, filter it and then either, let it dry out to make
verdigris or, split the solution into two equal amounts and to one part, add an excess of sodium carbonate which will precipitate
out copper carbonate and to the other, add an excess of sodium hydroxide solution which will precipitate out copper hydroxide.
Filter these out and you have a very fine particle-sized artificial malachite.
Lapis lazuli is usually mixed in with other rock and predictably, the purest is the most expensive. However, if you grind it
up into a fine powder then mix it with a mixture of wax and resin, let it cool down then each day, warm it up and massage it,
for about a week or so. Finally, warm it up and then massage it in water and the pure lapis lazuli powder will fall out and sink
to the bottom. This starts off fairly pure but as the massaging process continues, an increasingly large amount of the impurities
start to fall out as well. The best way to do this extraction process is to change the water at regular intervals so the first
batch is the purest then you have another batch and so on. Then, you can combine the batches that are of a certain grade so that
you are not mixing the purest with the most contaminated to make your paint.
The lapis lazuli relies on the fact that the lapis lazuli is more hydrophylic (water loving) than the impurities and so will
tend to fall out of the resinous wax mixture first. This differential of physical properties between the pigment and its impurities
is what all of these processes have in common.
The diagram on the right represents what happens to a ray of white light as it interacts with a pigment
particle in a transparent medium. At each interface between: air and medium; medium and particle; and,
medium and ground, there is an opportunity for reflection and refraction - within the pigment particle,
there is also an amount of absorption going on as well.
The incident rays starts off in the upper-right corner (as ray 1) and you can follow it around as it
makes its way into the medium (where it becomes ray 2), losing some light as a reflection off the
surface of the paint, into the particle (where it becomes ray 3), and where that ray starts to lose some
of its blue content, then is internally reflected, (becoming ray 4), passing out of the particle into the
medium (5) and finally out into the outside world whereupon, it can be observed by the viewer (6).
At each interaction with an interface, some of the original beam of light is lost and you can work out
how much by inserting values for the refractive indices of the two materials at each interface as well as
the angle of the incident ray. There is a page on Wikipedia about Snell's Law, Fresnel's equations
here. Basically, the greater
the difference in refractive index between the pigment and the medium, the greater the reflections at the
surfaces that the beam encounters.
Hopefully, the medium is colourless enough so that it doesn't absorb any appreciable amount of light as the
beam passes through it. The Predominant colouring process happens as the beam passes through the particles of
pigment.
Note that the absorption of the light is a process that happens gradually within the pigment particle and
that each time light enters a particle, some of it is reflected, back into the medium where it has the
opportunity either to return to the outside world or end up encountering another pigment article.
The paint sample on the right (pigments in acacia gum) shows both coarsely- and finely-ground pigment samples for:
Lapis Lazuli; and, Smalt.
These pigments are transparent when they are a single mass - smalt is alkaline (potassium) cobalt glass -
and as such, light is coloured as it travels through the pigment.
The coarse samples show what the pigment is capable of and what an artist would expect of the colours. However,
both smalt and lapis lazuli can be rather coarse, especially if you are painting fine detail, and they are applied
more easily of the particle sizes are finer. However, you can see what happens to them when they are finer.
Let's see what happens when the particle size is reduced.
This is a closeup of what happens when light falls onto a fairly coarsely ground pigment.
The light is reflected uncoloured from the surface of the pigment particles when it enters them, reflects
internally and then exits the particle having been coloured on its travels through the pigment, leaving the
paint layer and being observed by the viewer.
When the particles are smaller, the light can travel through more of them in the same volume of paint but
there are more surfaces to be reflected from and straight back out to the viewer before it has a chance to be coloured.
There are two main processes going on here so let us have a look at them in more detail...
This is natural cinnabar in its granular form. Its colour is quite intense and as a result of the large particle size, the light
incident upon it can travel a long way and be modified to a great extent before it encounters the other end of the particle along
with the opportunity to find its way back to the viewer.
These particles are around the size of granulated sugar so there is no way that you would paint with them - they need to be
ground - normally by sliding the muller over the slab so that the particles end up with an even size. However, there is an
interesting artefact of not doing that so, to illustrate a point...
This is the same sample of cinnabar but it has been ground by rotating the muller - not something that you would normally do. Grains
at the centre of the pile of pigment have nowhere to go so they are ground more finely whereas pigment at the edge will spread out and
end up as larger particles. You can see in the photograph that the pigment in the middle is ground so finely that it is almost an orange
colour (hue value of 13) whereas that around the edge is a deeper red (hue value of 0).
Normally, you wouldn't grind paint in that way as it is better to have a fairly consistent particle size. If you do have a wide range
of particle sizes and the medium that you are using has a low viscosity, the larger particles will tend to sink faster than the smaller
ones - if they are really small, they might even stay in suspension because of Brownian motion. The result of this would be that the
deeper coloured pigment would settle to the bottom and as you paint your way through the preparation, the hue would change.
This spectrum shows what happens to the wavelengths of light when they fall onto cinnabar that has been ground to different
particle sizes.
The deeper red is the larger particles, where all of the blue and quite a lot of green light has been absorbed; and, the lighter,
more orangey spectrum is from the smaller particles where the colour of the light is not changed very much within each particle and
there are more interfaces where light can be reflected back to the viewer, unaltered.
This effect has been used in paintings where a single pigment has simply been ground differently in order to produce two
different shades of the same colour. This has been done with azurite and also with malachite.
The reduction in saturation and shorter pathlength effects that you see here happen the most when there is a greater difference
in refractive index between the pigment and the particles - the amount of reflected light increases with this difference. So, if
the refractive index is increasingly similar, you would expect this effect to diminish and instead, the depth of colour to increase
or, as a minimum, stay very similar.
Ideally, the refractive index of the medium will be around the same value as the pigment. In this case, the light has
minimal chance to be lost in reflection before it has its colour changed by the pigment.
Another effect is that the light travels further into the paint layer before it is either scattered back to the
viewer or reflected back by the ground or underlying paint layers.
The consequence of this is that the amount of colour that is absorbed becomes more a function of the thickness of
the paint - with opaque paints, it doesn't matter how thick they are but with transparent layers, they get deeper, the
thicker they are.
This would not be a problem if the pigments were perfect - the magenta absorbed only green light, the cyan absorbed
only red light and so on. However, these are real-world pigments and magentas tend to absorb a bit of blue light and
cyans tend to absorb a bit of green light.
Many of us, as children, have wondered at the orange-coloured liquid in our mother's pantries that is labeled 'yellow
food colouring' because it is almost invariably a, orangey-red colour that miraculously turns yellow when it is diluted.
It does this for the same reason.
On the right, you can see some mango flavouring which is coloured orangey-yellow. You can see from the image on the
top-right that
it is the same liquid in two identical jars.
The image on the right is the same two jars with the same liquid in (you can tell from the shadows) but on the right,
where the light path is not as long, there is a shift in hue - a value of 0 on the left (pure red) to 46 on the right (40
is orange and 60 is yellow). This is the same liquid so why does this happen?
On the right, you can see the spectra of various sample points from the image. They show that whilst the food colouring
absorbs almost all of the blue light and transmits almost all of the red light, it also absorbs some green light.
As the path length (could be concentration instead) increases, the change in the amount of green light absorbed is greatest
so the most noticeable thing that happens is that with roughly the same amounts of red and blue light being seen, is a hue
change in an analogous way that as it did with the particle size in the cinnabar.
With regards to the real-life cyan paint that absorbs a little green light, when there is a lot of it in a paint, there
is enough green light absorbed for people to think that they are looking at blue paint. Similarly, the real-life magenta
paint that absorbs a bit of blue light will convince most people that they are looking at red paint.
The problem with this is that if you ask people what colours the three primary colours are in painting (subtractive
primaries) and they will reply; 'Red, yellow and blue' when they are really talking about what colours the masstones of
those colours are. In painting, like anywhere else, the three subtractive primary colours are yellow, magenta and cyan,
regardless of what people want to call them because, at the dilutions that you use them at, they are the colours.
If people are going to insist that they call them red, yellow and blue because they are the colours that they are in
the tubes, then you might as well call French Ultramarine, 'Black.'
The paint's medium can be almost anything that will:
- hold the pigment or dye so that it can be seen;
- allow light to pass through the pigment and then onto the viewer;
- preferably not react chemically with the pigment;
- be flexible enough for the support that it is on so that it doesn't crack over a short period of time; and,
- not shrink or expand significantly over time.
You need to be able to apply the paint to the surface where some sort of physical transformation will occur and the paint will be in a state
that will last. Either: you need to be able to reduce the viscosity of the paint by suspending particles in a relatively volatile liquid or by
dissolving the paint in a solvent; or, you need to have a physical process that makes the medium set. You can have both if the medium reacts to
solidify but is a bit too viscous without a solvent such as linseed oil. Examples of solvents are distilled turpentine and water.
So, what fits these requirements? Examples of some media are:
Media |
Medium |
Components |
Application Method |
Mobility Improver |
Solidification |
Time Between Applications |
Time to Finish |
Flexibility |
Longevity |
Colour Stability |
Chemical Stability |
Storage Form |
Notes |
Comments |
|
Oil paints |
Linseed oil Poppy oil Safflower oil |
Dilute with solvent |
Distilled Turpentine |
Solvent Evaporation then oxidation/ polimerisation |
Days |
Weeks |
Quite flexible although it hardens and becomes brittle with age |
Millennia |
Yellows a little but with a high enough PVC, not noticed |
Attacked by strong solvents |
Metal tube |
Is often supplied washed by acid (can attack some pigments) or alkali (causes soap formation and metal soaps leading to sweating) |
One of the earliest examples of painting with a medium is in some caves in Afghanistan where poppy seed oil was used as the medium. |
|
Encaustic |
Beeswax with Damar Resin |
Heat to melt |
None needed |
Cooling Down |
Minutes |
Hours |
a bit on the brittle side - only really suitable for stiff supports unless very thin |
Millennia |
Very Good |
Very Good |
Small Blocks |
Expensive to buy ready made paints but easy enough to make your own |
Encaustic dates back to Greco Egyptian times and before. |
|
Resins |
Damar Resin or Pine Resin |
Dilute with solvent |
Distilled Turpentine or Alcohol |
Solvent Evaporation |
Minutes |
Hours |
a bit on the brittle side - only really suitable for stiff supports |
Millennia |
Damar can yellow so high PVC needed |
Very Good but can be attacked by water or alcohol |
Made in situ from resin solution |
It is best to make this when you need it like some of the other media |
This dries very quickly if you use alcohol as the solvent. IPA is available as 99.9% pure IPA and is cheap. Use tends to be
limited to applying coloured washes on things like frames although there is no reason why it shouldn't be used elsewhere. |
|
Water Colours a.k.a. Tempera |
Acacia Gum Honey |
Dilute with solvent |
Water |
Solvent Evaporation |
Minutes |
Hours |
Quite flexible when thin |
Millennia |
Very Good |
Attacked by water |
Pans or Tubes |
This is used as water colours on paper but it can also be painted on other surfaces such as plaster and so on. When dry, the gum wets the surface of the pigment so the colours remain deep and saturated. |
Early use dates back to Egyptian times. Easy to make your own. Honey there as a humectant but some commercial watercolour suppliers
sometimes use glycerol which does not go solid. |
|
Egg Tempera a.k.a. Tempera |
Egg Yolk |
Dilute with solvent |
Water |
Solvent Evaporation |
Minutes |
Hours |
Quite flexible when thin and young although hardens with time |
Millennia |
Very Good. Does not yellow so blues are as good after half a millennium as they were when they were painted. |
Attacked by water |
Made in situ from egg yolks and water |
This is made each time it is used. The medium goes off if it is kept wet and it cannot be re-wetted if it dries out. With this medium,
it is the water that wets the pigment so when it dries out, the colours loose their depth - the minerals in the pigments are dry and are
effectively just held in place by the medium. |
This is one that dates back to works that are over a thousand years old. It has to be used when prepared as it cannot be stored for
more than a couple of days. The paint cannot be mixed on the work, it has to happen on the palette. It has to be applied in thin layers
because if it is applied thickly, it will crack. |
|
Glair a.k.a. Tempera |
Egg White |
Dilute with solvent |
Water |
Solvent Evaporation |
Minutes |
Hours |
Quite flexible when thin |
Millennia |
Very Good. |
Attacked by water |
Made in situ from egg whites and water |
This is made each time it is used. The medium goes off and doesn't last more than a day when wet unless refrigerated. It is made by whisking egg whites until the make a froth then leaving that overnight. The liquid that is left when the remaining foam is removed is the glair. This is then mixed with the pigment and water. |
This is one that dates back to works that are over a thousand years old. |
|
Casein a.k.a. Tempera |
Casein from milk |
Dilute with solvent |
Water |
Solvent Evaporation |
Minutes |
Hours |
Finished paint is inflexible and brittle |
Month |
Very Good. Does not yellow so blues are as good after half a millennium as they were when they were painted. |
Very Good |
Made in situ from milk curds, lime and water |
This is made from milk by curdling it with vinegar then mixing the separated curds with lime then adding your pigment. It will keep if refrigerated for up to two weeks and when it is dry, it becomes waterproof. |
Casein paints can be bought from some sellers in tubes although like all commercial preparations, the version that has to sit for months if not years in an art shop and still work when you decide to buy it and use it, is probably going to have a lot of other things in there. |
|
Distemper a.k.a. Tempera |
Gelatin |
Dilute with solvent |
Water |
Solvent Evaporation |
Minutes |
Hours |
Quite flexible when thin |
Millennia |
Very Good. Does not yellow so blues are as good after half a millennium as they were when they were painted. |
Attacked by water |
Made in situ from gelatin and water |
This is made each time it is used. The medium goes off if it is kept wet although it can be re-wetted if it dries out and is thin - add some water to it and heat it up by putting the palette over some steam while stirring it.
With this medium, like the egg tempera, it is the water that wets the pigment so when it dries out, the colours loose their depth -
the minerals in the pigments are dry and are effectively just held in place by the medium. |
This is one that dates back to works that are millennia old. It has to be used when prepared as it cannot be stored for more than a
couple of days wet. The paint can be mixed to some extent on the work but is easier if mixed on the palette. |
|
Acrylic paints |
PVA |
Dilute with solvent |
Water |
Solvent Evaporation |
Minutes |
Hours |
Quite flexible |
Only been around for decades |
Very Good |
Attacked by water |
Tubes |
Many of the same colours used in oils can be used here although water is the solvent and not spirits so not all are available |
PVA (Polyvinyl Acetate) is a product of the 20th century chemical industry and therefore its longevity is completely unknown. Being
an ester, it can be attacked by acids or alkalies. Many of the colours have titanium white added to them giving the paints an overall pale look. |
|
Fresco |
Plaster |
Dilute with solvent |
Water |
Solvent Evaporation then crystalisation |
Minutes |
Hours |
Brittle. Only suitable for rigid surfaces such as brickwork |
Millennia |
Very Good. |
Attacked by anything that attacks plaster such as mould when wet |
Made in situ from plaster and water |
This is made each time it is used. The medium goes off quickly and sometimes pieces need to be completed in small areas. The medium
is alkaline so a lot of pigments are unsuitable. With this medium, like the egg tempera, it is the water that wets the pigment so when it dries out, the colours loose their depth -
the minerals in the pigments are dry and are effectively just held in place by the medium. |
This is one that can last millennia under the right conditions. |
|
Glaze |
Glass |
Suspend in Water |
Water |
After water evaporated, fire in a kiln to melt the medium |
Minutes |
Days |
Brittle. Only suitable for rigid surfaces such as metal and ceramics |
Millennia |
Very Good. |
Attacked by Alkali |
Powder from manufacturer |
Biscuit fired items are either dipped in glaze or have glazes painted on them. The articles are then fired at a higher temperature where the clay vitrifies and the glass powder melts, completing any chemical reactions to make the pigments. |
Often the colour of the pre-fired glaze can be quite different to that of the finished article. Colours such as Cobalt Blue (Silicate) start off as Cobalt Oxide which is grey. Also, you can mix a pigment such as cobalt oxide in with the clay which you then use decoratively on the article (such as dividing your stoneware clay into two, mixing pigment in one half, assembling a cylinder with one half with pigment then putting it on the wheel to make a spiraled pattern) and then glaze over it with a clear glaze to bring out the colour. |
|
Medium |
Components |
Application Method |
Mobility Improver |
Solidification |
Time Between Applications |
Time to Finish |
Flexibility |
Longevity |
Colour Stability |
Chemical Stability |
Storage Form |
Notes |
Comments |
|
Pigment Volume Concentration is a measure of the ratio of pigment to medium in terms of volume. It is volume that is
important because some pigments are denser than others.
Some pigments are light and fluffy (French ultramarine and carbon black spring to mind) and other are quite dense (lead
white and cinnabar, for example). The important thing about the ratio of pigment to the medium that it is in is the volume.
If you have a lot of medium, there might not be enough pigment in it to have the covering power that you need. However, if
you had a lot of pigment in there, the paint would be more like putty or plaster and would not flow onto the canvas very well. If
you diluted such a high PVC paint with thinner, when that evaporated off, there might not be enough medium there to hold the
pigment in place.
In short, PVC is a fairly useful guide on how to mix paints for a consistent outcome.
With paints with media such as egg yolks, you should use a PVC of one to one so, a pile of pigment and add enough egg to be
an equivalent volume. Then, you can add as much water as you need to but when the paint dries out, there will still be a PVC
of 1:1
We add solvents to our paints because without them, the paints would not be easy to apply to the artwork - they lower the viscosity of the paint, hence their other name - 'thinnners'.
A solvent effects a lowering of the paint's viscosity in two ways:
- firstly, it makes the medium less viscous; and,
- secondly, it increases the distance between the pigment particles.
In order to lower the viscosity of the medium, it needs to be miscible with it or dissolve it. In the case of water colours, the medium is usually acacia gum so the water that you add to it dissolves the gum, making it flow better than the solid lump of paint that you have in the pan - pigment held together that is applied dry is in effect clay pastel. In the case of oil paint, the solvent dissolves in the linseed oil, lowering its viscosity quite substantially. Additionally, it has to be chemically inert as far as the paint is concerned oterwise it would make changes that the artist would not be able to control.
The second effect - increasing the distance between pigment particles now takes effect because the volume of the paint is that much greater for a given amount of pigment so, with greater distances between pigment particles, they are flow over each other easier.
Once the paint has been applied, the solvent needs to evaporate, leaving no trace of it behind - anything that is left behind needs to be a solid or a compound that will turn into a solid - usually be oxidation in the case of oil paints. Anything left that does not become solid will destroy the integrity of the painting. As the solvent evaporates, the medium shrinks, bringing pigment particles closer together and increasing the paint's viscosity again.
So, to summarise, a solvent has to:
- Dissolve in the medium;
- Evaporate from the paint in a reasonably short period of time;
- Leave no practical trace that it was there; and,
- Not react chemically with the paint or surface.
Hydrophobic Media
Solvents for oil/wax/hydrophobic media tend to be some form of volatile spirit - turpentine, essential oils and so on.
Turpentine is made from the destructive distillation of resin that has been collected from a plant - usually pine resin. The resin is heated up until the heat literally makes the resin fall apart - cracking it (in the chemical sense) - and the resulting volatiles are collected and called turpentine. From the fact that turpentine is obtained by heating up the source material to a temperature that will decompose it, the condensate is very likely to contain a number of chemicals that whilst volatile enough to turn into vapour at cracking temperatures, still have a high boiling point and therefore not be that suited to adding to paints to thin them as these lower-volatility compounds will be left in the paint.
It is the nature of distillation that if you simply flash-distill (that is, do it quickly) a mixture, you are going to get a product that has a range of molecular weights in it and therefore different volatilities. This 'gum spirit' is sold by Roberson. If you want to make sure that you only have the volatiles in the liquid, you need to re-distill it, taking care this time not to get what is called 'splash-over'. The light ends are your distillate and the less volatile compounds stay in the still's base. The light ends are sold by Winsor and Newton as 'English Distilled Turpentine'.
Lavender oil is steam-distilled from lavender plants and is used extensively in the perfume industry for things like washing powder, air care products and so on. It has the property that insects don't like it (many essential oils are pathological responses from plants to insect damage so they are there to cause damage to insects to reduce damage to the plant and the insects have evolved to not like the smell of lavender amongst other things). It is thought that Jan van Eyck used lavender oil as a thinner because it is a volatile oil so I obtained some for this test from 'naissance' (English Lavender) and 'biOrigins' (Spike Lavender).
By its very nature, the steam distillation process can pull over many compounds that have quite high boiling points so if a mixture is obtained by steam distillation, quite a high proportion of it can have boiling points that are undesirable for the artist who just wants a solvent for thinning paint or cleaning brushes. Fractional distillation can do that but steam distillation cannot guarantee it.
The predominant compounds in the lavender oils for the English lavender are: Linalool (around 30%); and, Linalyl Acetate (around 33%). For Spike Lavender, they are: Linalool (around 50%); Eucalyptol (around 25%); and, Camphor (around 13%). So, you can see that they will probably have different properties when it comes to rates of evaporation.
So, we have two variants each of two hydrophobic solvent products that can be obtained by artists for thinning down paint to use in oil paintings. How well do they perform?
Determination of Relative Evaporation Rates
Determining absolute rates of evaporation is probably not that meaningful as there are going to be differences between each artist's studio and differences within each artist's studio for different times of day, time of the year and so on. More meaningful is the relative rates of evaporation so I decided on a method that would compare each so whilst the absolute results might vary according to air temperature, draught and so on, you can still tell just how much faster or slower one solvent is when compared to another.
I used aluminium make-up pots without the screw-on lids, 3.6cm in diameter and 2cm tall, numbered them and weighed them. I then accurately weighed in between 1 and 2g of each solvent. I also included two additional pots, one with acetone and one with rain water - the intention being that these would be familiar points of reference. I weighed them every quarter of an hour to start with and then every half hour on a balance that measured to 0.001g. The liquid in each produced a layer of liquid in the order of 1mm deep so to start with, the weight loss was a representation of how much mass can be lost through around 10 square centimetres of surface where migration of the liquid to the surface is unhindered. The experiment was performed in a domestic kitchen without any forced draughts. The room temperature was 22C - 23C throughout.
I then tested the four hydrophobic solvents for residues by weighing in approximately 1.5g of each solvent and letting them evaporate at room temperature, weighing them then putting them in the oven for 30m at 80C.
Results:
The turpentines evaporated steadily and relatively quickly, surprisingly at around the same rate as the water but the lavender oils were very slow and eventually had to be blown by a fan for around 6 hours before they had finished evaporating at room temperature.
I had included acetone and water firstly because most people are familiar with them but also, because I thought that they would bracket the other liquids - acetone being fastest and water being the slowest so it came as quite a surprise to find that even though the acetone behaved as suspected and the water performed as well as the turpentine, by far the worst was the lavender oil, both in terms of its rate of evaporation and the residue left behind.
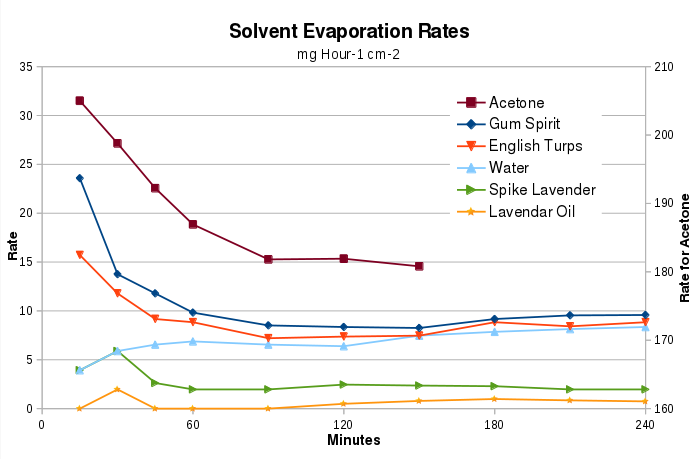
Crucible | |
1 | |
2 | |
3 | |
4 | |
5 | |
6 | |
Solvent | |
English Distilled Turpentine | |
Gum Turpentine | |
Lavender Oil Lavandula Angustifolia | |
Spike Lavender Lavandula Latifolia | |
Acetone * | |
Rain Water * | |
Brand | |
Winsor & Newton | |
C. Roberson & Company | |
naissance | |
biOrigins | |
Lucemill | |
Nature | |
Initial Evaporation Rate † / mg cm-2 hour-1 | |
16 | |
24 | |
1 | |
5 | |
200 | |
6 | |
Established Evaporation Rate / mg cm-2 hour-1 | |
8 | |
9 | |
1 | |
2 | |
180 ‡ | |
7 Þ | |
Residue at Room Temperature | |
<1% | |
5% | |
5% | |
3% | |
<1% § | |
<1% | |
Residue at 80C for 30m | |
<1% | |
3% | |
2% | |
2% | |
<1% | |
<1% | |
Residue - Physical | |
V. thin film, resin-like, dry. | |
Thicker film, resin-like, tacky | |
Viscous, oily | |
Grease, smears | |
none | |
none | |
Residue - Smell | |
None | |
Slight 'pine' smell | |
Slight, Sweet | |
Slight, Sweet | |
Olefinic, 'P'-Side (C3) | |
None | |
* | Acetone and water added to table to show rates for familiar liquids for comparison. |
† | Initial rate (approximate) reflects presence of light ends except in acetone. |
‡ | Acetone's evaporation rate was so high that its temperature was around 4C lower than the other samples. |
Þ | Water's latent heat of evaporation was so high it was around 1C lower than the other samples. |
§ | Some drops of water remained after the acetone had evaporated having condensed from moisture in the room - this soon evaporated. |
|
Conclusion
Ideally, you need a solvent that will increase the volume of your paint sufficiently to allow you to apply it in the way you want, and then to evaporate, leaving nothing behind. Additionally, it should do this not so fast that it dries out whilst you are still painting with it but not so slowly that it is still there after your linseed oil has finished polymerising - reducing the volume of the paint layer after polymerisation would lead to a weakened paint layer.
Turpentine evaporates off at about the right rate for this process and the English Distilled Turpentine leaves no appreciable trace behind it. Whilst the Gum Turpentine evaporates at pretty much the same rate, it does leave between 3% and 5% residue in the form of a resin behind which is, in effect a low molecular weight version of the resin it came from - ie, colophony or pine resin. In order to reduce this to acceptable levels, all that needs to be done is to distill it so that these heavier ends don't end up in the final product and this is, what the English Distilled Turpentine is.
On the other hand, the lavender oils did not evaporate very quickly - at less than a fifth of the rate of the turpentine - and as they went on, the evaporation rate deteriorated considerably. Finally, both the lavender oil and the spike lavender oils both had around two per cent oil that was a heavy oil in one case and a grease in the other - neither of these being acceptable in oil paints.
So, if you want to add a solvent to your oil paint to make it paint better, go for the English Distilled Turpentine. If you can't get that then the Gum turpentine will do but whatever you do, don't waste your money or time on the lavender oil, wether it is ordinary or spike, it just takes too long to evaporate.
Hydrophylic Media
Hydrophylic media are basically all of those that will, as the name suggests, use water as the solvent. Water is water but it is not the water that is the problem,
it is what is dissolved in it that is the problem.
Tap Water
Water from your tap has plenty of contaminants in it because the source of that water is often a reservoir or a river - note that reservoirs are filled by rivers.
All sorts of contaminants get into rivers such as nitrates from fertiliser draining from fields, calcium from dissolving rock and so on. If you live in a hard water
area, there will be more calcium in the water than in soft water areas.
The amounts of these contaminants in tap water are quite low - the calcium as calcium carbonate in my tap water is between 50 and 60ppm by weight,
in other words, a litre has between 50 and 60mg of chalk dissolved in it.
So, whilst boiling gallons of water over years in a kettle will leave a scale on the inside, adding a few millilitres of tap water to your water
colours isn't going to turn your artwork into a sea of scum. However some things in your water can react with pigments with undesirable effect or speed up the
degradation of your artwork.
So, what alternatives are there to tap water and what was available centuries ago?
Deionised Water
Deionised water also called 'demineralised water' is tap water that has been through a couple of ion-exchange columns. One removes cations (things like ammonia, metal ions
such as copper, calcium, magnesium and so on) and one removes anions (things sulphate, carbonate and so on). The resulting water is free from these inorganic salts but the columns
don't remove organics (unless they react with the resin of the columns).
So, deionised water has the salts removed from it but not the organics.
Distilled Water
Distilled water has been boiled and the steam collected and condensed - usually in a piece of glass apparatus. This can have some salts in it if the boiler part of
the apparatus has been running too long without the water being exchanged - some are designed to so replace this constantly so buildup and scaling doesn't occur as much - and droplets have been carried over with the vapour.
The head of the still is usually an anti-splash head,
designed to reduce the level of misting. The best designs for anti-splash heads are based upon the cyclone so that liquids are separated out centrifugally (the largest one of
these that I have seen was around 2m across as part of a petrochemical plant that I used to work in the lab for).
However, volatile organics and volatile salts can still make their way across into the distillate so again it is not perfect.
Tumble-Dryer Condenser Water
Like distilled water, this has evaporated by warm air from the damp clothes and the air/water vapour then cooled down by the dryer's heat pump to below its 'dew point' so that it condenses out.
This is then pumped into a container so that you can tip it away when enough of it has collected.
Even though it has not been boiled as such, it has in effect gone through the distillation process and as such is very low in salts and so on.
However, even though it is distilled water, there are usually bits of lint in it and whilst you can filter these out and use the water as ironing water - it is not going to clog up your iron with
salts - there are also other organic chemicals in there.
In chemistry, there is a process called steam distillation and it is used to distill from mixtures chemicals that would normally decompose if heated up to their boiling points. The ratio of water
to the other chemical is a function of their molecular weights but you can get oils and very small quantities of light waxes to go over in the distillate - this is what is in fabric conditioner.
Therefore, whilst these contain chemicals that are alredy part of the washing process and will not interfere with your steam iron, they are not part of the art process and therefore would be foreign
to, say, water colours or other water-solvent-based arts
Therefore. appealing as this might sound as a potential source of water for art, it is not advised.
However, volatile organics and volatile salts can still make their way across into the distillate so again it is not perfect.
Rain Water
Rain water has got to be pure, hasn't it? Well, no. It is made by evaporating sea water, the vapour then travels upwards by usually a mile or so and then it condenses out
and it falls as droplets through around a mile of air and then hits your collecting device.
The clouds are sufficiently far up for any problems of splashing not to exist although sea water can be whipped up into a spray that is carried upwards so that is no guaranteed.
Additionally, there are tornadoes and water spouts (think of raining fish or frogs) which carry water and other contaminants upwards by large distances that can include as far
as the clouds. And, then, there are sand storms - remember that Saharan sand and other material is carried across the Atlantic ocean to South America.
Next, it falls through the air and has the opportunity for around 5 minutes to pick up dust, particulates (algae, pollen, fungus spores and so on), along with soluble gasses such as oxides of nitrogen and carbon
dioxide along with ammonia if it is in polluted air, before it is collected. If it has been raining for a short while, the contaminants that are not in the whole of the air such as
industrial pollutants like ammonia and so on, will have been scrubbed out of the air by the rain that has already fallen - think of the air looking clearer after a storm.
Finally, it hits your collecting device and can pick up pollutants and dust that is on that although, again, if you let it rain for a bit first, a lot of that should be
washed out of the way before you start collecting.
Once you have it in storage, if you let it stay in strong sunlight, there is bound to be at least one algae cell in there that will use the available nitrogen compounds
and carbon dioxide to grow and you might well end up with a green tint to the water so keep it in the dark.
So, which is better? As an analytical chemist and an artist, I would go for the rain water stored properly and if that runs out, use tap water.
In short, the ground is the surface that you draw or paint upon and the support is what holds it in place.
Support | Ground | Notes |
Stone / Brick |
Plaster |
The finished plaster surface is extremely absorbent. Usually, pigment is mixed in
with the final plaster layer to produce the painting which is called 'Fresco.' In this case, the pigment
environment is alkaline and only a subset of the normal set of pigments can be used. Artists' colourmen can
advise on which pigments are and are not suited to this environment - one being L. Cornelissen.
Alternatively, the final plaster layer can be sized in order to seal it and then pretty much any type of paint
can be used on the surface. |
Porcelain |
- |
Usually, it is the surface of the porcelain that is painted on directly with paints that
are in effect either powdered coloured glass or powdered minerals in powdered glass. Sometimes a slip made from
clay with powdered minerals is used for painting pictured. This is all then fired at kiln temperatures and
various chemical reactions take place, giving the final colours.
Alternatively, the surface an be treated as any other porous surface by treating it with size and then whatever
colours in whatever medium you feel like if you are not going to fire it again.
|
Papyrus |
- |
Papyrus is already sized to a large extent by the glue-like chemicals in the plant's sap
and there is no need to add anymore to it. Traditionally, it is painted on with inks made from soot and paints that
are made from powdered rocks although Egyptian blue and vermilion (artificial cinnabar) were used by the Egyptians.
On papyrus, the paint tends to stay where you put it. |
Vellum / Parchment |
- |
Paint and ink is applied directly to the skin. It is not porous and the ink or paint just sits there
until it dries. Any grease from your skin will stop the paint or ink from staying there and the surface generaly has to be prepared by going over it with pumice or some other fuller material. |
Paper |
Size |
Paper for painting is usually made from waste cotton fibre with size in the water already so
you don't have to size it yourself. You can paint on more size if you like, depending upon what you are doing.
Smooth - hot ironed - paper is better for drawing with inks as the loose fibres in the rough paper stock will catch
on the nib/quill. |
Wood |
Size / Gesso |
If you do not at least size the surface of wood, it bleeds anything that you put on the surface.
With size, your work still sits where you put it but if you put gesso on there as well and then sand and scrape it, you
have a nice, flat surface upon which to work. If you need to do punch work, then size the wood, stick
on some cloth such as muslin or linen then apply gesso, smoothing with sand paper and then scraping as above. You can
also use gesso to produce pastiglia
which can be left or fashioned with various implements. |
Canvas |
Size then Gesso |
Canvas will rot if you let linseed oil come into contact with it (so I am told) so first apply
size and then coats of gesso and smooth as you want. Canvas is flexible so it is best not to make it too thick as the
gesso will crack. Also, take into consideration the instability of canvas if you are considering using pastiglia in
the painting by not making it too thick, otherwise it might simply crack and drop off. |
Metal |
Gesso (optional) |
Metal is very stable and is used both for paintings that are going to be fired like ceramics
and also for painting on with oils. Without a layer of ground to reflect the light, transparent paint virtually glows
when compared to paint that has been applied to areas that have paint underneath - the light passes through the paint
and instead of most of it being lost in the process of scattering, almost all of it is reflected back towards the
viewer producing a substantially different effect. This method was used for a while to paint jewels from medieval
times. |
|
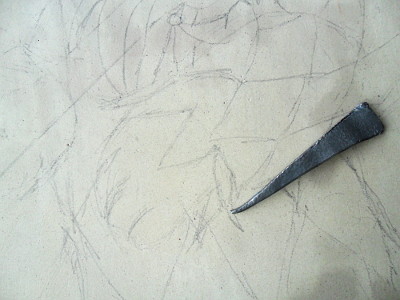
Gesso ground on solid oak support.
|
|
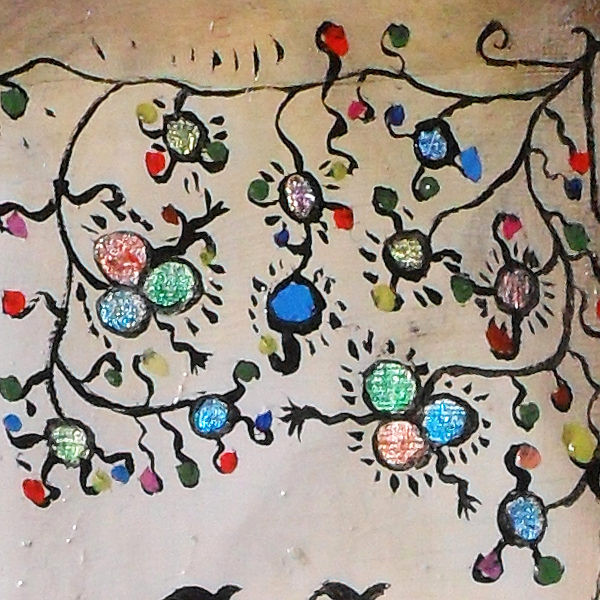
Transparent paint on silver leaf.
|
|
4
Colour
We see colours in paints because the paint absorbs or otherwise prevents our eyes from seeing certain wavelengths. You can represent colours by using pure light or mixtures of light and a useful tool for predicting what will be seen when mixing paints can be made from this.
Move the mouse over the little CIE diagrams at the start of each section below to see that CIE diagram on the right.
Eyes. The iron-shaped CIE diagram on the right is a representation of hue and saturation that your eyes can see - the area for a given set of circumstances is called a 'gamut'. How dark or bright a colour is is not covered by the diagram because it is really a three-dimensional diagram of which this is a slice through the middle - white and black being at the hue centre, above and below the diagram.
Wavelengths. The wavelengths of the pure colours are around the curved edge because they represent a hue that can be reproduced by using light of a single wavelength.
Notice that there are no wavelength numbers along the straight edge at the bottom.
Absorption Wavelengths.
Along the straight edge, between blue at 400nm and red at 700nm is a line of hues that need two colours of light to reproduce them or, from white light, can be represented by an absorption peak with a single wavelength. In the middle of the 'iron', there is a grey area and if you draw a line from the colour you see, through the spot at the centre, to the other side, you can see the wavelength of peak absorption that can be used to produce that colour.
For example, an aqueous solution of potassium permanganate looks purple and if you look on the straight line where an absorption peak corresponding to 530nm (yellowy green) appears, you will see the purple reproduced. The actual permanaganate absorption is a number of peaks that mass together but their centre is around 530nm.
Saturation. The further away from the edge that you get, the less saturated the colour. Taking yellow as an example,
A laser would produce a single wavelength so it would be located at the edge of the diagram. Two LEDs can also convince you
that you are seeing yellow light even though there is no yellow light there at all. If the wavelengths are close enough to
each other, it can appear almost as saturated as the laser light. A yellow ink or dye only has to absorb blue light and that can
appear quite saturated. The more light that you can see, the brighter it is and that is one of the things that is confusing
to most people.
Screen. Your monitor screen does not use pure colours to produce the colours that you can see - these would not be bright enough and your eyes adjust to a lowering of saturation by automatically compensating for it so a compromise is made of a useful brightness against a useful purity.
One feature of the CIE diagram is that if you want to produce an intermediate hue by mixing two colours, the resulting hue and saturation will appear on a straight line between the two colours you started off with. By starting off with the optimised red, green and blue that your monitor can produce, you end up with the small RGB triangle on the right.
Printer. Following on from the RGB triangle, the CYMK triangle is based upon the even less saturated colours that your printer uses and as a result, has an even smaller gamut. The only reason that your printer seems to produce such saturated colours is that you are used to looking at images that are so dull and your eyes compensate for this to some extent.
Interestingly, some people claim that you can duplicate any colour there is just by using a palette of White, Magenta, Yellow, Cyan and Brown (black is not permitted although white is - note that you get a reasonably proficient black by mixing the brown with the cyan) and then they will match any colour from a colour print - of course, the gamut is so small that all they are doing is matching the printed colours and not the colour of the original subject. Single pigment paints give the best colours as you can see from the chart.
Lasers. Nominally, lasers will produce a single wavelength of light - in reality, it is a bit wider than a single wavelength but for most uses, this doesn't matter too much.
As the laser light is pure, it will sit at the edge of the chart. However, our eyes don't differentiate between pure colours and slightly broader ones and we will think that a light source that is centred around a particular wavelength is just as saturated up to a point, even though it is not. For this reason, we can look at the light from a red and a green source, mixed together and think that they are yellow. You can do this yourself with a red and green laser and even though there is no yellow light there, it looks yellow.
Early Colour Pigments. These are some of the colours that were available at the beginning of the 15th century - except for Egyptian Blue, that one is artificial and they had forgotten how to make it by then.
Terre Verte is an earth pigment - like the Siennas, Ochres and Umbers - but the main colouring agent is chromium oxide. For the reason that it is primarily an earth mixture that is dug out of the ground, there is a lot of variation in the colours you get for this pigment - saturation and brightness, but the hue is basically the same.
Common Mixtures. One thing you might notice from the above selection of colours is that there is little in the way of yellow-greens and purples. The latter was solved by mixing together rose madder and lapis lazuli (or, for a less pure version, rose madder and azurite) for the purple variants. This was easy because they don't react with each other.
The yellow-greens (or 'Vergaut') is a different story. Orpiment is Arsenic Sulphide and Verdigris and Malachite are copper compounds. Copper Sulphide is black so if you are using a medium that doesn't seal in the particles of pigment, hydrogen sulphide can be released from the Orpiment and will attack the Malachite or Verdigris so, if you are using Orpiment, the green that you mix it with needs to be Terre Verte.
However, Lead Tin Yellow II is all right with copper so either Verdigris or malachite is a mixture that will last. In oils, the medium seals the pigments in so it doesn't matter as much but it is still better to play safe - these mixtures are to be found in Jan Van Eyck paintings which date back to the early 15th Century so they do last but the passage of time is inevitable and chemicals do react in any medium so it is better to play safe.
Effect of Particle Size on Mixing
On the right are paint cross-section diagrams that represent what happens with paints with different sizes of opaque pigment particles imagine that the painting's viewer sees the painting from the top of each diagram.
We take two hypothetical colours in two particle sizes where each of the particles is spherical.
In diagrams one and two, the particle size is small whereas in three and four it is large.
In diagrams one and three, the pigment is white whereas in two and four, it is black.
Paints one and three should look the same as each other as they have the same pigment to volume ratio and paints two and four should
look the same as each other as they have the same pigment to volume ratio. For a given shape, it doesn't matter how big the particles are
because they will always have the same particle to void ratio - in the case of paint, pigment to medium.
If you mix equal volumes of one and two together (5), you will get a mid grey because you can see equal areas of each pigment.
If you mix equal volumes of two and three together (6), paint two will tend to fill in the interstitial gaps in paint three and therefore
dominate it. In short, the pigment with the smaller particles will tend to cover the pigment with the larger particles because the smaller
particles fill in the gaps between the large particles more effectively.
This effect is more pronounced, the greater the difference between the particle sizes.
With transparent paints, this does not happen because this effect relies upon the light being reflected back to the viewer as soon as it
has interacted with the pigment particles at the surface.
This sort of thing can be tolerated by the artist making their own paints (see image on the left of larger pigment particles shown by
palette knife on the grinding slab) but if you are going to manufacture paints that are going to sell on, you need to make sure that your
particle sizes are pretty much the same from paint to paint - something that would make paints from real pigments such as malachite, azurite, lapis lazuli, smalt, orpiment and realgar quite troublesome because the artist would have to have a better idea what was going on.
All images and original artwork Copyright ©2020 Paul Alan Grosse.